5 Steps to Identify Green Sand Metalcasting Defects
- Hill and Griffith
- Oct 2, 2018
- 6 min read
The procedure of identifying, diagnosing and minimizing or eliminating crucial casting defects is important for a foundry to run in a low-cost, and high-efficiency mode.
It can disprove incorrect conventional wisdom and show that unexpected methods are actually the best way to move forward.
To do this, there are three keys to remember:
Focus on identifying a casting defect on the basis of its appearance.
Be aware of the interactive nature of foundry processes and variables.
Use rigorous experimental design methods to study complex causes of defects.
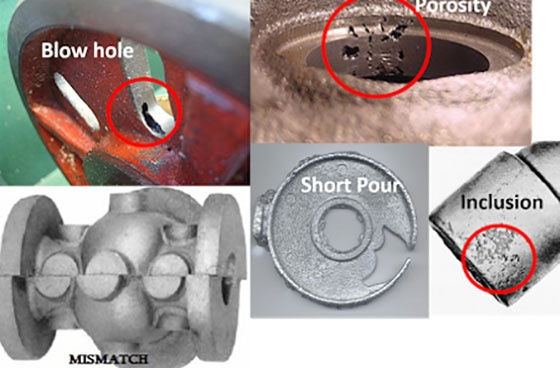
(Thanks to Green Mechanic for the image. You'll find more about metalcasting defects at their site.) Foundry personnel can be quick to label a defect cause based on a cursory examination. Terms such as slag defect and cold shut are part of the defect process. The International Casting Defect Atlas gives a specific code/category to defects based on appearance. It also suggests foundry personnel be aware that most casting defects are due to the interaction of several process variables rather than one factor, such as temperature or gating system design. This allows the foundry engineer to design experiments to capture the complexity of the defect cause. AFS Corporate Member CWC Textron Foundry (Muskegon, Michigan) used this process of casting defect categorization, identification, cause determination and defect reduction to address an issue with camshaft castings.
The defects began occurring after an upgrade from manual pouring of molds to automatic pouring of molds. Auto pouring systems are considered to be safer reliable and efficient compared to manual systems.
However, in the early tests comparing castings from manually poured molds to castings from automatically poured molds, the casting scrap rate was always significantly higher for the automatically poured molds. Other variables, such as pouring temperature and transfer time impacted the scrap rate.
This gray and ductile iron foundry has been producing alloy and ductile camshafts for many decades. All molds are produced on a horizontally parted, high pressure, tight flask, green sand, automatic molding line. Flasks on this molding line move on a conveyor to the pouring area.
Primary melting is done with a cupola. The base iron is held in a channel induction furnace. MgFeSi is added to a large tundish ladle as it is filled from the induction furnace. In manual pouring, the ladles were filled from the tundish ladle and inmold inoculation was used. The main sources of casting scrap are were simply listed as “dirt” or “slag.”
Now, an automatic pouring system has replaced the manual pouring ladles. The equipment was acquired and installed to make mold pouring safer and more consistent from mold to mold. The automatic pouring system ladle holds significantly more iron than the manual pouring ladles. During the transition to automatic pouring, the manual pouring area was kept intact.
Whenever molds were poured using the automatic pouring system, the camshaft castings exhibited a high frequency of cope-side inclusion defects. These defects were small, but deep enough to cause the castings to be scrapped. To solve the slag, pinhole, and dross defects, CWC Textron worked through a proven step-by-step approach.
FIVE-STEP PROCESS
Details of each of the steps followed are shared below. There may be changes in the methods and tools specific to an individual foundry.
Step 1 – Identify the Defect
Foundry personnel have a tendency to identify a defect based on cause like slag defect or sand inclusions. While this is an acceptable method, after the diagnosis is done, the International Atlas of Casting Defects recommends that unknown defects be classified based on appearance rather than cause. Using the photos and descriptions given in the atlas, the foundry decided these defects appear to be G121 (inclusions) or B123 (pinholes) (Figures 2 and 3).
The key advantage of this classification system is that foundries are allowed to have multiple labels for the defect and leaves it more open to investigate different causes for the defect.
Detailed optical micrography confirmed the defects were dross and pinholes related to magnesium vapors. It is essential to verify the casting defects through such detailed analysis, prior to exploring cause and remediation steps. In addition to optical microscopy, SEM (scanning electron microscopy) along with spectroscopy was used.
Step 2 – Experimental Design
When the castings were poured with the manual ladles, the frequency of inclusion defects was very low. When the castings were poured with the automatic pouring system, the frequency of inclusion defects was very high. Also, it was observed that on a same day at the same time, under similar sets of green sand parameters, frequency of the inclusion defects was still higher with the automatic pouring system compared to manual pour. This would suggest that metal and molding sand were not primary causes of defect. For this reason, no trials were run with modified base iron metallurgy or modified molding sand properties.
Instead, CWC focused on studying the pouring temperature, pouring time and method, Mg-treatment and inoculation method (tablet in manual pour, in-stream in automatic pouring unit). Table 1 summarizes the key variables and factors considered. Detailed fractional factorial design experiments were designed based on well-known statistical methods. Most foundry casting defects are caused by interacting variables (for example, low pouring temperature + specific chemistry).
Factorial design is a tool that allows experimentation on many factors simultaneously. In this case study, researchers ran 2 level factorial design with three factors requiring a total of eight runs. Three factors considered in the experiment were: ladle type (regular ladle and insulated ladle), temperature (lower pouring temperature and higher pouring temperature) and pour cup (D-shaped cup: conical cup with one side flat- and offset-basin cup). Detailed factorial design/experimental design is provided in Table 2.
Step 3 – Gating Design and Filtration Review
Often, foundry personnel jump to modifying the gating system when they observe slag/dross defects. While turbulence in the gating system may be an important factor, the gating system is one of the few constants in the multi-variable production environment of the foundry. Since this gating system worked well in the manual pouring system, no major modifications were proposed. It was noticed that the cross-sectional area of the sprue base and runner was very large and the pouring time was controlled by manual pouring operation. It is strongly recommended that computer simulations of solidification and flow are conducted to review the performance of the gating system. Oxidation of iron and formation of inclusions will likely increase as the velocity of the metal increases. Slowing down the flow and keeping the gating system full may show a reduction in surface inclusions.
Filters should be considered an “insurance” policy rather than the main function of keeping external slag and dross away. Inefficient dross removal practice can lead to filter blockages, quickly leading to misruns and slow pours. Filters are often considered “flow modifiers” as significant dross in castings are related to turbulence in the runner and ingate system.
There are two aspects of filter sizing: the primary sizing is related to ensuring that the filter does not act as the choke. The standard rule of thumb is the cross-sectional area of the filter should be at least 4-6 times that of the choke.
The secondary sizing requirement is related to filter capacity, or the volume of ductile iron that can be passed through the filter prior to blockage. Typically, this capacity is around 20-40 lb./square inch of filter.
Filter pore size can be classified as fine, medium and large. In most applications, medium or large openings are preferred. The filter supplier can provide appropriate sizing sheets for particular castings and filter types.
In the case of this project, the filter was considered adequate for the specific application. Excellent guidelines are available for sizing and placing filters in gating system.
Step 4 – Preliminary Trials
It is important that trials be conducted with just a handful of variables. Proper experimental designs are required to ensure interaction effects are captured (effect of pouring time, temperature and chemistry together, for example). Pours were grouped by heat, and at least 10-20 molds were poured per heat. It is important to measure and document all variables related to the casting.
Step 5 – Production Trials
Production trial volumes and details are important in a quality assurance program. Production trials for automotive applications typically require thousands of castings. Some foundries might review data for a whole shift or for several heats to ensure repeatability and reliability of quality. Data tracking includes pouring temperatures, pouring times, microstructure, chemistry, lab tests related to mechanical properties, and other information.
During the preliminary and production trials, the pouring was done automatically but the metal transfer was manual (by forklift) and, therefore, took more time, resulting in a higher than normal temperature loss. Following the five-step process, key recommendations to reduce the defect included:
Increase the pouring temperatures.
Control pouring temperatures (by improving ladle insulation).
Reduce magnesium additions.
Increase the bismuth addition after MgFeSi treatment.
Improve the automatic pouring ability to pour in the center of the cup.
Reduce velocity and turbulence of the metal in the mold.
Operate the automatic pouring system with fully automatic ladle filling.
Thanks to Global Casting Magazine for this article.
Metal Foundry News - From Foundry Management and Technology Magazine
Comments